Core science: cosmology
To achieve its primary scientific goals, Euclid will map the web-like spatial distribution of matter in the Universe, (Fig 1) whose properties are influenced by the expansion of the Universe and the nature of the dark matter. Simplified, a stronger expansion causes less ‘clumping’ of matter and thus a smoother large-scale structure (LSS). Smoother structure may also be expected, to a lesser extent, depending on the properties of dark matter itself, uniquely connecting cosmological scales with micro-physics. By comparing the statistical properties and morphology of the LSS with simulations and theoretical models, we can infer how the Universe expanded, study how matter structures formed and, ultimately, predict the Universes fate.
The Euclid mission will do this primarily using two complementary methods: weak gravitational lensing and galaxy clustering.

Weak gravitational lensing – using shapes for a projected map of the matter structure
Weak lensing uses a specific aspect of General Relativity: mass bends light rays similarly to optical lenses. Consequently, the matter structure impacts how we observe the image of far-away galaxies by deflecting the light from the galaxies on its way to us (see Fig 2 on the left). This process distorts the galaxies’ images as if we looked at them in a fun-house mirror. The distortions encode how much matter the light rays encountered on their way to us and can thus be used to map the matter distribution.
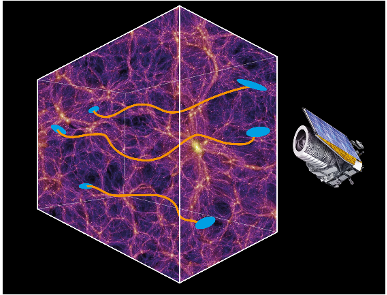
Illustration of weak gravitational lensing. The light rays (orange) of far-away galaxies (blue) are deflected multiple times on their way to the observer. This distorts the observed shapes of the galaxies. [Image credit: Springel et al. (2005)/ESA/L.Linke]
There are two complications in this approach, though. First, we do not know the original galaxy shapes. Therefore, we average the observed shapes of many galaxies. The averaging cancels out the original galaxy shapes, and only the effect due to the matter distribution remains. For precise results, we need to average over as many galaxies as possible. Euclid will obtain shape measurements of 1.5 billion galaxies over a survey area of 15 000 square degrees, spanning a third of the sky (see Table 1).
The second complication is that the shape distortions are tiny, typically only a few per cent, so galaxy shapes must be determined with high accuracy. Euclid is ideally equipped for this since it observes far outside of Earth’s atmosphere and can therefore obtain images at optical wavelengths that are almost as sharp as the Hubble Space Telescope.
Weak lensing has one disadvantage: the shapes are impacted by the sum of all the matter encountered by a light ray on its way to us. Hence, we can only map the matter structure as projected onto the sky. The 3D distribution of the LSS is not directly accessible, although some information can be recovered by dividing the galaxies into subsets by distance. However, more precise distance estimates enable another powerful probe: galaxy clustering, the second primary probe of Euclid.
Galaxy clustering – using galaxies as tracers for matter
Galaxies are luminous tracers of the matter distribution, and as such describe how density varies on different scales due to both initial conditions and the growth of structure (see Figure 3 below). However, to reconstruct their true 3D distribution, we need to measure galaxy distances. Luckily, the expansion of the Universe offers us a tool do to that, through spectroscopic observations, i.e., measurements of the energy distribution of their light as a function of wavelength. Spectral features due to the emission of well-known chemical elements (like hydrogen, oxygen, or magnesium) are found in galaxy spectra, shifted to larger wavelengths compared to their laboratory value. This is the so-called cosmological red-shift, which is induced by cosmological expansion and is directly proportional to the galaxy distance from us.
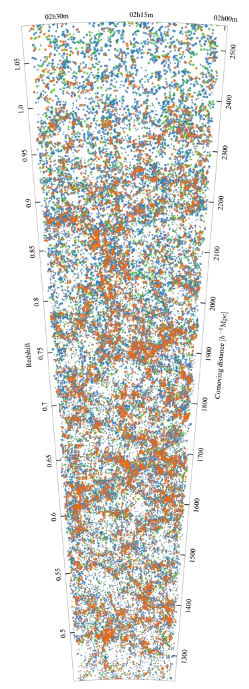
The clustering of ~60,000 galaxies reconstructed by a large redshift survey covering a significant fraction of cosmic time (redshifts z between 0.5 and 1.1). Galaxies with different physical properties (here their intrinsic colours) trace large-scale structure in different ways, with red objects highlighting the highest density skeleton of the 3D distribution [Image credit: VIPERS collaboration 2017]
Thus, the 3D spatial distribution of a sample of galaxies on the sky can be reconstructed through a spectroscopic redshift survey. Euclid will do this for around 35 million objects, reconstructing a 3D map of the galaxy distribution over an enormous volume in the distant Universe. The inhomogeneity on different scales in these maps will be then quantified through specific statistics of the density fluctuations and used to constrain model predictions, which directly depend on the key ingredients of the theory describing the Universe (as, e.g., the density of dark and standard matter).
Euclid will measure the value of these ingredients to unprecedented precision, using weak lensing and galaxy clustering independently and also in combination. This will allow us on one side to obtain the highest possible precision, while on the other hand checking, through their comparison, for subtle systematic errors that may affect each of the two probes.
Property | Weak lensing galaxy sample | Galaxy clustering galaxy sample |
---|---|---|
Redshift range (z) | 0–2 | 0.84–1.88 |
Redshift measurement and accuracy | Photometric, better than 0.05(1+z) | Spectroscopic, better than 0.001(1+z) |
Number of objects | 1.5 billion shapes | 35 million 3D galaxy positions |