In the first half of July we will launch a Falcon 9 rocket into far Earth orbit, carrying Euclid – a 1.4-billion-Euro precision instrument to explore the far universe. So why is the European Space Agency spending this budget on this space mission and not, say, 200 million pieces of avocado toast, for example? In fact, there are too many great answers to this question. This blog post will only focus on one: the main goal of the mission – to increase our understanding of the dark universe.
What can Euclid tell us?
From the Mezopotamians to Douglas Adams, humans have been able to answer the ultimate question about life, the universe, and everything to varying degrees of satisfaction. We are privileged to live in a time, where we have many tools of understanding at our disposal. We have access to the microcosm through Earth-shattering experiments like the Large Hadron Collider, we can peer into the fabric of time with gravitational waves detected by LIGO, and we can behold the opaque wall at the end of our observable universe with ESA’s Planck probe. This way we can try to address the big questions. How did the universe start? How will it end? And how come we can exist in it at all?
To answer these fundamental questions, we have to look no further than as far as we can see. Since light travels with a finite (as opposed to infinite) speed, it needs a lot of time to cross great astronomical distances. For this reason, we are always not only looking into the distance, but also peering into the past. So if we make great maps of the distant universe, they are not only maps in space, but timelines of the history of the universe, that enable us to study its evolution. If we can understand its evolution well enough, perhaps we can infer how things began, and how things will end. But this is where the going gets rough.
The mysteries of the dark universe
Albert Einstein formulated his theory of gravity at the beginning of the 20th century. Since then, hundreds of physicists around the world have been working day and night to prove his equations wrong. So far, they have all failed. Why are we trying so hard to prove him wrong? Because if you do observe the distant universe, it seems that gravity is messing with us. There are two opposing forces in play.
On the one hand, there seems to be “too much gravity” – already in the 1930s, Fritz Zwicky noticed that giant clusters of galaxies seem to rotate too quickly. Later, in the 1970s, Vera Rubin provided compelling evidence that confirmed the same problem existed in galaxies themselves.
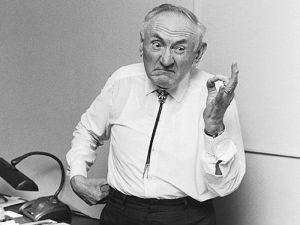
If what we can see were all that was there, the galaxies and clusters of galaxies would fly apart! This is because anything that rotates without flying apart needs a centripetal force to hold it together. On a merry-go-round, you hold on to the handlebars. The stars in a galaxy are held together by the gravity of the mass of the galaxy itself. If there is not enough mass, there is not enough centripetal force. Bye-bye galaxy. In order for these giant cosmic objects to exist, and rotate at the observed speeds, there needs to be an unseen mass. We have pointed all kinds of telescopes and probes at it and we can’t see anything. No light is emitted by this stuff. It doesn’t absorb any light, and it doesn’t reflect any light. At any wavelenght. All we can “see” is its gravity. So we call it dark matter.
On the other hand, there seems to be “not enough gravity”! Even though no-one has managed to prove Einstein’s equations wrong, his interpretations of the equations have been
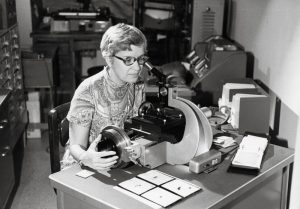
shown to be wrong on several occasions. He even admitted himself that he made a mistake in insisting that the universe is static. You see, we’ve known for a long time that the universe is actually expanding. But in the 1990s, a big team of scientists – led by Adam Riess and Saul Perlmutter – proved that distant galaxies are not only flying away from us, but are going faster and faster with time – the expansion of the universe is accelerating! What is driving this acceleration is a huge mystery – a tough nut to crack. But it isn’t the first time that the universe has accelerated. By observing the distant universe, we see evidence that the first memory of our universe is of an epoch of extreme accelerated expansion. We call it cosmic inflation and we think it lasted only a tiny fraction of a second. Cosmologists postulate that it was driven by a kind of “energy field” with a brief existence that disappeared a long time ago. We believe that a similar kind of field may be driving the accelerated expansion today. We have never detected this field directly. We only see it’s strange, reverse gravity. We call this field dark energy.
Dark matter and dark energy are the greatest mysteries in fundamental science today. Together we sometimes refer to them as “the dark universe”. Whoever finds out what they are, is bound to win the Nobel prize and be forever written into the annals of the history of science as the dark universe hero.
What are we looking for?
So how do we make these maps of past time and distant space? And when we make them, what do we look for in them? How do we find clues about the dark universe?
Even though we can’t see dark matter or dark energy, we can see their effects on things we can see. By observing the bright objects in the universe, we can try to understand all the gravitational influences that dark matter and dark energy exert upon them. In order to see billions of light-years into the distant universe and billions of years into the past, these objects of course need to be extremely bright to be picked up by our telescopes. So, individual stars won’t do. So we make maps of the locations galaxies, each made up of billions of stars, and their properties. This way we can study how galaxies have been pulled together – by dark matter – and pushed apart – by dark energy – through cosmic history.
The Euclid space telescope will be capable of detecting galaxies far enough to be looking 10 billion years into the past, to a time not yet 4 billion years after the Big Bang. The satellite will carry two different instruments, which will not only let us detect the galaxies, but also measure several of their properties.
Galaxies cluster into huge structures on the sky
Euclid’s near infrared instrument will let us measure how quickly distant galaxies are moving away from us. Believe it or not, this relative speed of galaxies can tell us about both dark energy as well as the nature of gravity! There are two main contributions to the recession velocity of distant galaxies: the “cosmological” and the “peculiar”. We will measure their combination for each galaxy by looking how “redshifted” galaxies seem. We will only be able to separate the two components by using clever statistical methods.
BAO and what cosmological velocities can tell us about the universe
‘BAO’ are not just delicious buns in Chinese cuisine. It’s also a cryptic acronym that stands for Baryon-Acoustic Oscillations. These BAO look like enormous bubbles that permeate the universe. Each BAO bubble measures almost exactly 50 million light-years across. They are not real bubbles of course. They are imprints from the early universe in the pattern of how galaxies are scattered through space. And they make excellent cosmic rulers – standard rulers, if you will. Because we can calculate their sizes very precisely from simple physics of the early universe, they are excellent markers that help us measure cosmological distances from their apparent size on the sky.
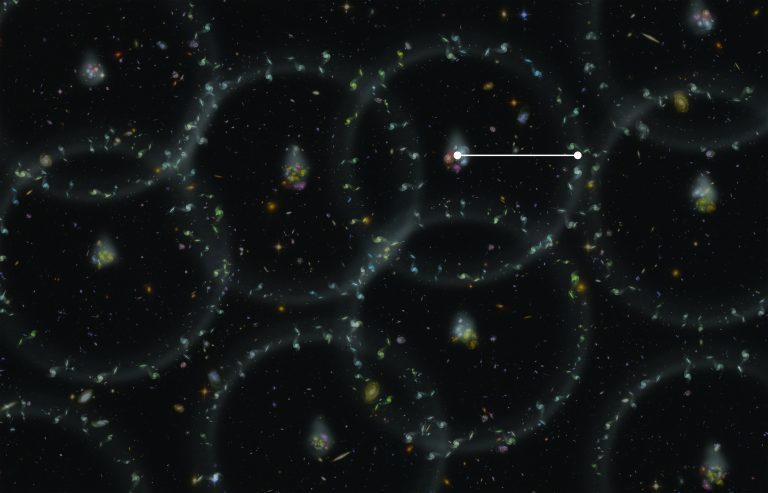
Baryon Acoustic Oscillations from SDSS-III – Illustration Credit: Zosia Rostomian (LBNL), SDSS-III, BOSS.
The strongly dominant, “cosmological” component of the recession velocity of distant galaxies is due to the expansion of the universe. Here we have to be careful – you can never measure the relative velocity between us and something that is billions of light-years away! The reason for this deserves a separate (future) blog post and has to do with the difference between General and Special Relativity. However, for the sake of argument, you should know that it does look like the universe is pulling the galaxies away from us at seemingly measurable speeds, which equal a significant fraction of the speed of light – up to then 70% for the galaxies Euclid will see. So, if the universe were expanding with a constant speed, if galaxy B were twice as far as galaxy A, galaxy B would look like it’s moving twice as fast away from us as galaxy A.
This means that measuring both the apparent “cosmological” speeds and the distances of very far away galaxies can help us understand how the universe is expanding. Or rather, how the universe has expanded through cosmic history. Using the Euclid infrared instrument, we will measure both, the apparent sizes of BAO on the sky, and the redshifts of the galaxies that make up their bubble shells. This technique for understanding the expansion history of our universe, and in turn, the nature of dark energy has been proven in the past. Surveys of distant galaxies like the 2dF, 6dF, SDSS, Vipers, BOSS, and recently eBOSS have found the BAO bubbles and used them to put constraints on what kind of “gravitational pushing” dark energy could be capable of to expand the universe. In the future, Euclid will not only find more of these BAO because of the larger volume of its survey – it will specifically look much deeper and therefore much further into the past!
Redshift space Distortions and what peculiar movement of galaxies can tell us about gravity
The other component to the recession velocity of distant galaxies is small compared to the cosmological – with values much smaller than 1% of the speed of light. This component is due to so-called “peculiar” velocities of galaxies. This simply means that galaxies orbit around large masses in their neighbourhoods and generally move around according to the nearby gravitational fields. As we have said, redshifts are proxies to how quickly galaxies are moving away from us. But if you accidentally assume that the entire velocities of galaxies come from the expansion of the universe, if you forget that galaxies move around their nearby gravitational potentials, you will see strange things in your cosmic maps. Things like apparent “fingers-of-god” will appear, as well as “great walls”. These are what we call Redshift Space Distortions. But they are not just strange artefacts in our maps. They are much more valuable than that – they can tell us about the nature of gravity. If Einsteins theory of gravity is correct, the artefacts have to look just so. Any deviation from what the theory predicts could indicate strange kinds of matter, energy or even gravity!
Redshift space distortions in data and in computer simulations obeying Einstein’s theory of gravity.
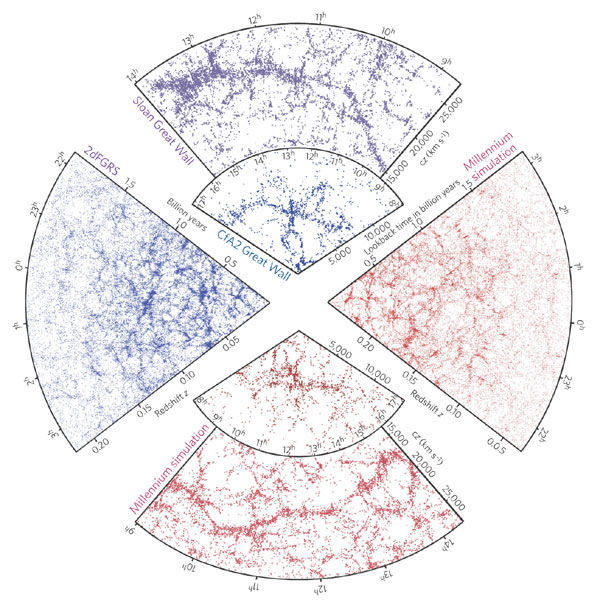
Massive objects are gravitational lenses
But so far, we have only talked about Euclid’s infrared instrument. Euclid will carry another instrument that will be capable of picking up the visible light from distant galaxies. It turns out that the visible light is very good for detecting the shapes of distant galaxies. Why do we care about the shapes? The light from distant objects doesn’t only carry information in its colour (and therefore redshift). Any distortions in the paths of photons of light carry information about the kind of universe they have traveled through. We can try to detect distortions in the shapes and structures of the images of distant galaxies. And these carry the clues of the photons’ paths. You see, light always finds the shortest path through space. So in flat, undistorted – empty! – space, it travels along straight lines. However, just like for an airplane traveling along the surface of a sphere, in curved space, the shortest path may not be a straight line. So any curvature in space will distort the images carried by photons that travel through it.
Just like a billiard ball on a rubber sheet, the presence of mass curves space. So if a photon passes by a massive object, its path becomes deflected, it strays off the straight line to keep its travel as short as possible. This means that as photons of light travel through the universe, the massive objects they encounter imprint their presence on the images carried by the photons. This effect is known as gravitational lensing. Massive objects act as giant gravitational cosmic lenses. The gravity can come from things that we see, like stars, galaxies, and black holes. But it can also come from things we don’t see – like dark matter.
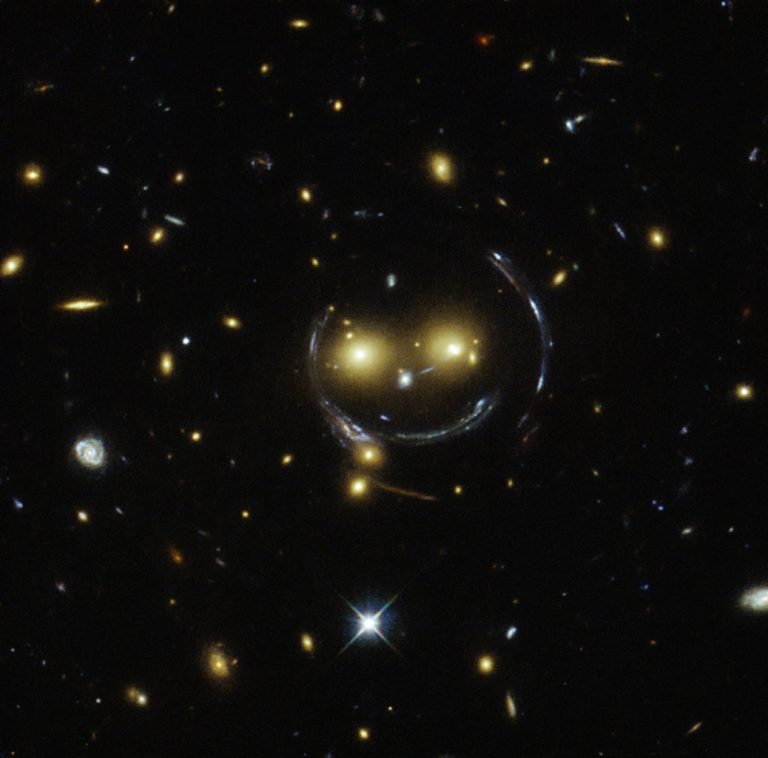
Strong gravitational lensing of images of distant galaxies. This incidental arrangement of foreground (lens) galaxies and the arc-like distorted images of background galaxies was first captured by the Hubble Space Telescope.
The Euclid visible light camera VIS will enable us to capture the images of over a billion galaxies. The photons carrying these images will have spent up to 10 billion years traveling through the expanding universe full of structures of visible and dark matter.
It is very rare that we can see the distortions of the images of distant galaxies by eye. When we do, we call it strong gravitational lensing. But the much more common occurrence is weak gravitational lensing, which is present over the entire sky. This is why we also call it cosmic shear, only a very slight shearing of all galaxy images. But without knowing distances, we can only make the maps two-dimensional. Luckily, we know that Euclid will have a tool to measure distances in its near infrared instrument. The near infrared instrument will measure redshifts, which can be converted into distances, if the cosmic expansion history is understood, and if any small peculiar distortions can be ignored. We loose some precision by ignoring the small, peculiar velocities, but it’s worth it. This way we can use a kind of cosmic tomography: split our galaxy images into distance slices and study the contributions to cosmic shear through time and space. This way, with distance information and advanced statistical methods, we can extract a three-dimensional map of dark matter by looking at how much and into which direction the images of galaxies are sheared and flexed.
In the past, cosmic shear has been used as a proxy to make just such maps of dark matter by galaxy shape surveys like the CFHTLens, and recently the Dark Energy Survey. Unlike Euclid however, these surveys looked at only fractions of the extra-galactic sky we can see. Euclid will survey the entire sky that is not blocked out by our home galaxy, the Milky Way.
Putting it all together
Combining the information from both surveys (the visible and infrared) will present us with unprecedented scientific and “big data” challenges. This will be the first time a single experiment will attempt to combine both, the maps of galaxies and those of dark matter, on such a large scale. For this reason, hundreds of scientists around Europe and the world have spent decades diligently calculating the specifications for the satellite and the instrument that will give us the best window into the dark universe. We have also spent decades preparing our methods of data analysis and information extraction for the upcoming challenge. They will enable us to make amazing maps of both, galaxies and dark matter in the universe. These maps will not only help us understand gravity by examining the structures they contain, they will also enable us to study cosmic history by looking back in time. They will reveal the great cosmic web on which galaxies glisten like dewdrops on a spiderweb. A spiderweb, whose shape in space and time contains clues about the past and the future of our cosmos. So if you like puzzles, big or small, keep an eye out for Euclid and its mission to understand the dark universe!
A computer simulation of the cosmic web of dark matter and galaxies. This simulation was created especially for Euclid – it is the largest simulation of the universe to date! (Image credit: Joachim Stadel)
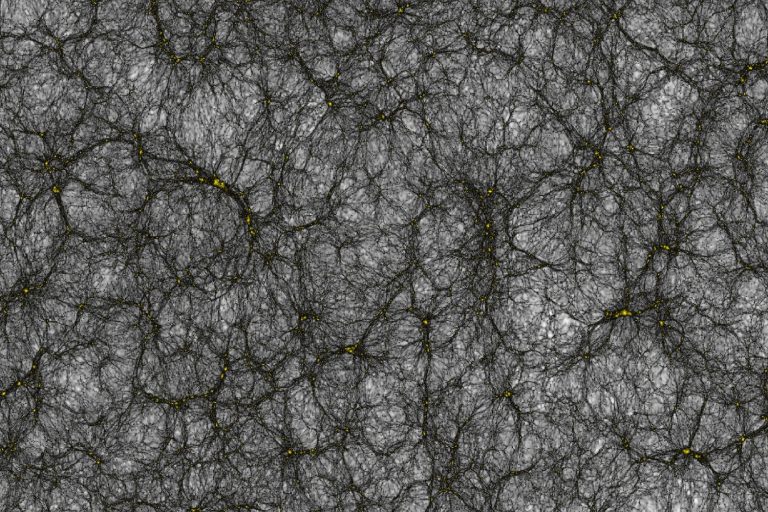