Euclid’s core mission is to measure cosmological parameters to unprecedented accuracy with the aim to enable astronomers to decide between different cosmological models. Euclid’s data is taken by two exceptional astronomical cameras: VIS and NISP.
The current Euclid mission originates in two previously proposed concepts for ESA cosmology missions called DUNE and SPACE. These independent proposals used the complementary effects of weak gravitational lensing and galaxy clustering as diagnostics for measuring cosmological parameters. During ESA’s selection process for new science missions in their “Cosmic Visions” programme DUNE and SPACE were merged into one new concept, Euclid.
With these techniques, Euclid needs to do three things: wide-field imaging with high resolution visible imaging for weak lensing; near-infrared photometry for distance measurements of galaxies; and determine the exact location of a subset of galaxies using spectroscopy. This combination sets Euclid’s technology as well as where in space it has be located and which galaxies to look at.
To reach its core cosmological science goals, as well as to enable a multitude of “legacy science”, Euclid will have to map out about 1 billion galaxies, which corresponds to a deep wide field survey of about 1/3 of the sky. It will do so with a combination of a wide-field telescope , and scientific instruments, using optical elements to turn them into images or to split up the light according to energy and create spectra, as set by the requirements previously set by science.
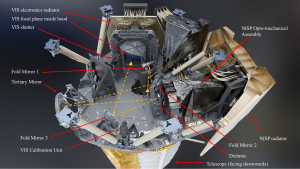
The capabilities needed for Euclid result in two separate instruments using different technology. One is the Visible Imager or VIS for short, an imaging camera in the visible wavelength range, and the other the Near-Infrared Spectrometer and Photometer NISP.
The unique challenge in the instrumentation for Euclid is that instruments must be extremely stable and the the path that light travels to impact on the detectors changes as little as possible. The second challenge, of course, is that everything must operated reliably for half a decade or more in the harsh environment of space, with radiation, and cold vacuum. To achieve these goals, the instruments have been designed to be as simple as possible to accomplish the task at hand, and are constructed from materials that change as little as possible under mechanical and thermal stresses.
Imaging in visible light: VIS
The light falling on to the Euclid mirrors is reflected several times and then ultimately arrives a coated glass plate, the so called “dichroic”, a coated glass plate which splits the beam into visible light and infrared light. These beams head to two separate instruments, VIS and NISP. So let us look at these in some detail.
The VISible instrument (VIS) works in much the same way as the camera inside your smartphone or digital camera – only it has about 100 times as many pixels and it will be 1.5 million kilometers away in space.
The camera is composed on 36 charged-coupled devices (CCDs), each one having 4000 x 4000 pixels. As a comparison an HD TV only has about 1920 x 1080 pixels. That means that each picture that Euclid takes with the VIS camera is 278 HD TVs worth of data – and it takes one of th
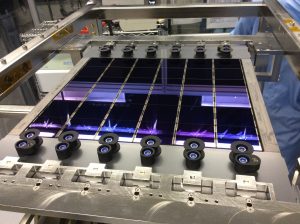
ese pictures about every 10 minutes continuously for more than 6 years. Each pixel is 12 millionths of meter square. When a photon of visible light strikes a CCD, it creates an electrical charge, and the intensity of charge is proportional to the intensity of the photon. This electrical charge can be then read out of the camera.
The wavelengths of light over which the VIS camera takes pictures are very similar to those that the human eye is sensitive to, VIS’ observed range is from 550 to 920 nanometers. The only difference being that VIS cannot see in colour only in black and white. Colour information will be provided by lower-resolution ground-based data taken in multiple wavelength bandpasses.
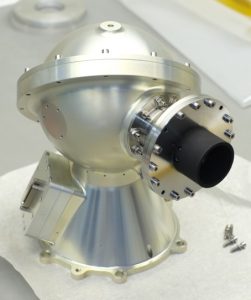
The large number of pixels in the VIS camera means that the area of sky the pictures can cover is huge for an astronomical camera. In a single shot the VIS camera will be able to take a picture of 0.56 square degrees of sky – this is about 2.5 times the size of a full moon in every snapshot.
But the VIS instrument is – and needs – so much more than just the pixels. To support these pixels is a thermo-mechanical structure and electronics which convert electrical impulses from the camera to usable science images. There is also a tiny light source called a Calibration Unit that can shine a blank reference picture onto the camera to make sure we can convert electrical quantities into physically meaningful units. To power and control the camera there are more electronics that take power from the solar panels
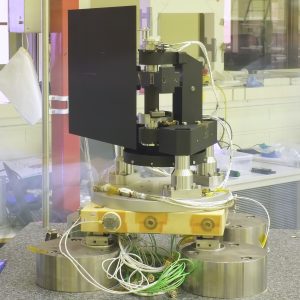
on Euclid, designed with multiple redundancy to be failsafe, so if they break for whatever reason there are backups to take over. Finally there is a shutter mechanism than can open and close the light path to the VIS detectors, much like the shutter in a normal camera just much larger. We need a shutter so that when Euclid is moving we can close the shutter and stop taking pictures. The shutter must open and close tens of thousands of times throughout the lifetime of the mission at a very precise speed.
Only with all these components the images taken by VIS will be precise enough and stable enough to be used for weak gravitational lensing measurements. Taken togther, Euclid’s VIS camera weighs about 113 kg and will be one of the largest digital cameras ever launched into space.
The sky in the near-infrared: NISP
The Near-Infrared Spectrometer and Photometer instrument, in short NISP, aboard Euclid will observe the sky at wavelengths between 950 and 2000 nanometers, using the near-infrared light passing through the dichroic plate described above. NISP will can take direct images in three different near-infrared bandpasses, but can also split up the light with a dispersing “grism”, a combination of a prism and a grating. This splits up the light of every celestial source along its wavelength, or energy, and produce a intensity spectrum.
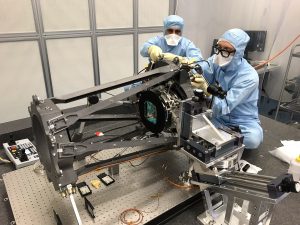
This versatile capability, which is a very different approach to the single bandpass, high-stability design of VIS, is enabled thanks to NISP carrying exchangeable filter and grisms that can be rotated into the science beam. Four grisms are located in one wheel, three filters and a light-tight shutter position in a second wheel.
The light that NISP observes of such long wavelength that is invisible both to the human eye – which is sensitive from very blue 400 nanometers to red at about 700 nanometers – and also to conventional silicon detectors such as those used in VIS or in digital cameras, which stop being sensitive around 1050 nanomenters. The 16 detectors in NISP are provided by NASA and each have about 4 million pixels. They are made from mercury-cadmium-telluride, “HgCdTe” in short, a material that can be tuned to sensitivity at different infrared wavelengths. The NISP chips are sensitive up to about 2300 nanometers. Similar detectors have already been used for decades and also in recent space mission. They are currently flying onboard the Hubble Space Telescope and will be used in the James Webb Space Telescope.
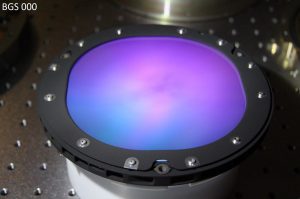
The light-sensitive part of the NISP focal plane, tiled with these 16 detectors, has a size of 15 x 15 cm. Together with correspondingly large optics to observe a wide field on the sky – the same size as VIS -, mechanical structure, movable wheels, and also a calibration lamp this results in a “camera” more than 1 meter in length. This is substantial given that NISP can have a maximum mass of 95 kg for optics and detectors and 60 kg for its data-processing electronics. For this reason the design required use of rather exotic materials: extremely light and hard Silicon Carbide for most of the mechanical structure, and aluminium, titanium, and an alloy called Invar for all wheels and holders of lenses, filters, grisms and detectors.
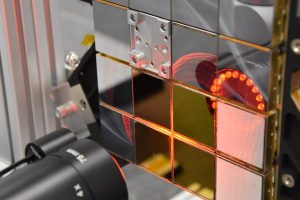
If NISP wanted to downlink all observed data back to Earth, it would exceed any bandwidth limit by Euclid’s high-gain antenna. So beyond the mechanical and optical parts, NISP also not only has to record the data observed but also carry out massive data processing on board to reduce the volume of the initial data taken down to one single image per point in the sky it observed, reducing the data volume to 290 GBit per day. This sets the computing power that has to be on board, and which in turn has to be able to operate with not more than 200 W power at any time – that is less than the power consumption of a current TV or computer.
For both VIS and NISP we will go into more detail in future posts. At the time of writing the VIS and NISP instrument development teams are fully engaged to complete their flight hardware and to start assembling their instruments. Most of this work will take place during 2018 and 2019. Followed by extensive tests before both instruments are integrated, together with the telescope, into the Euclid spacecraft.
Tom Kitching & Knud Jahnke